![]() |
|
Biography
Viktor Sverdlov received his Master of Science and PhD degrees in physics from the State University of St.Petersburg, Russia, in 1985 and 1989, respectively. From 1989 to 1999 he worked as a staff research scientist at the V.A.Fock Institute of Physics, St.Petersburg State University. During this time, he visited ICTP (Italy, 1993), the University of Geneva (Switzerland, 1993-1994), the University of Oulu (Finland,1995), the Helsinki University of Technology (Finland, 1996, 1998), the Free University of Berlin (Germany, 1997), and NORDITA (Denmark, 1998). In 1999, he became a staff research scientist at the State University of New York at Stony Brook. He joined the Institute for Microelectronics, Technische Universität Wien, in 2004. His scientific interests include device simulations, computational physics, solid-state physics, and nanoelectronics.
Spin-Dependent Trap-Assisted Tunneling in Magnetic Structures
Energy efficient spin-transfer torque magnetoresistive random access memory is reshaping modern microelectronic circuits by introducing non-volatility not only in memory, but also in logic. Boosting the sensing margin by improving the tunneling magnetoresistance ratio (TMR) is a pressing challenge currently under intense investigation. As the dimensions of magnetic tunnel junctions are reduced, a single-electron nature of trap-assisted charge transport starts to prevail.
The Coulomb interaction leads to the repulsion of the charges on a trap. This repulsion results in a Coulomb blockade when the double occupancy of the trap is prohibited. The Coulomb blockade is the cause of strong correlations in electron hopping. Electron transport then occurs in stages consisting of an electron jumping from the source electrode to the trap, followed by the electron escaping the trap to the drain electrode. Because Coulomb repulsion is a purely classical interaction, the electron tunneling through the trap represents an example of classically correlated charge transport.
The Pauli exclusion principle forbids two electrons with the same spin projections to occupy the same quantum state on a trap. This results in yet another correlation effect on transport through the trap. In the case of ferromagnetic contacts, the electron impinging on an electrode from a trap has a larger probability of being accommodated by the electrode if its spin is parallel to the magnetization of the electrode. The spin correlations result in the Pauli blockade. This spin-dependent trap-assisted hopping between the two ferromagnetic electrodes (Fig. 1) produces large magnetoresistance modulation.
Usually, strong spin dephasing and spin relaxation level the spin-dependent properties off. In the case of trap-assisted spin-dependent tunneling only, however, the spin relaxation is detrimental (Fig. 2). Surprisingly, strong spin dephasing enhances the difference between the maximum and minimum currents. Interestingly, the current maximum is achieved when the drain magnetization is not parallel to the source. The TMR value at strong dephasing is larger than the value for direct tunneling. At the same time, spin dephasing reduces the noise level (Fig. 3) at the maximum current, rendering the potential of spin-dependent hopping for practical applications.
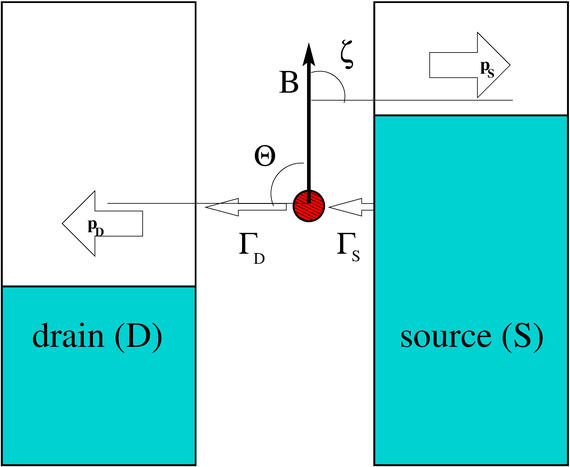
Fig. 1: Trap-assisted tunneling between the source and drain ferromagnetic electrodes.
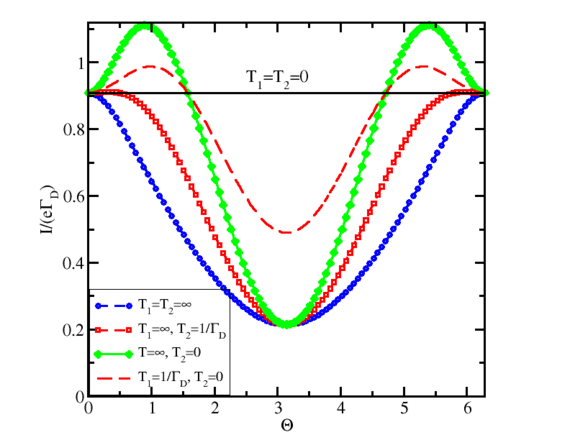
Fig. 2: Trap-assisted tunneling current between the source and drain ferromagnetic electrodes as a function of Θ, the angle between the two magnetizations. The magnetic field direction is in the direction of the source magnetization.
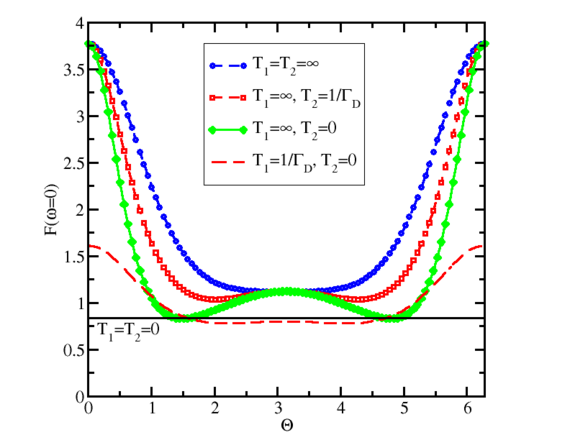
Fig. 3: Effect of spin relaxation and dephasing on shot noise at spin-dependent trap-assisted hopping between the ferromagnetic electrodes as a function of the angle between the source and drain magnetizations.