![]() |
|
||||
Biography
Mario Bendra was born in 1993 in Steyr, Austria. He received his BSc degree in Mechanical Engineering from the University of Applied Sciences Upper Austria in 2017. After an exchange semester at the University of the Sunshine Coast, Australia in 2018, he received the degree of Diplomingenieur in Mechanical Engineering from the University of Applied Sciences Upper Austria in 2019. Mario joined the Institute for Microelectronics in October 2020, where he is working towards his doctoral degree focusing on developing and implementing advanced computational approaches to simulate and optimize spin-transfer torque magnetoresistive memories. |
A Multi-Level Cell for Ultra-Scaled STT-MRAM Realized by Back-Hopping
Spin-transfer torque magnetoresistive random access memory (STT-MRAM) is one of the most promising candidates for future nonvolatile memory technologies. An MRAM cell consists of several layers, including a CoFeB reference layer (RL) and a free magnetic layer (FL), separated by an MgO tunnel barrier (TB), forming a magnetic tunnel junction (MTJ). To increase the perpendicular magnetic anisotropy, the FL, typically composed of two CoFeB layers and a thin metal buffer, is interfaced with a second MgO layer. Elongating the FL and introducing additional MgO layers in the middle of it allows for a further increase in the perpendicular anisotropy, while also reducing the cell diameter. However, if the length of the FL falls below a certain threshold, we predict an undesirable switching of the second FL section, which gives rise to a back-hopping effect. This, in turn, leads to a writing error in the magnetization state of the free layers. However, this effect can subsequently be utilized to realize multi-level cells (MLCs) by adjusting the polarization of the TB in our favor. Considering this, an MLC based on more than one MTJ is proposed to further enhance memory density. However, the implementation of an MLC requires carefully tuned characteristics of each MTJ to achieve different resistances and switching currents, leading to the formation of multiple stable states.
We employ a fully three-dimensional (3D) finite element method (FEM) based modeling and simulation approach to investigate the switching behavior of ultra-scaled MRAM cells with a composite FL. Fig. 1 shows the magnetization trajectories, when switching from (a) AP to P and (b) P to AP under different configurations of FL length. For the AP to P case, successful switching is achieved, regardless of the FL configuration. However, switching from P to AP is only achieved, when the FL configuration is symmetric and the length of the FL segment is larger than 5 nm. In Fig. 2, we report on switching realizations of the FL with the TB with increased interface spin polarization inserted between the two parts of the FL. A higher TB polarization leads to a higher spin current flow, so that the torque acting between the two FL segments is stronger than the one between the RL and the first part of the FL. Fig. 2(b) illustrates the back-hopping effect. As reported in the inset, the structure with two FL sections with thicknesses of 3 nm each continuously transitions between the different states, as long as the current is applied. In Fig. 3 we report on switching realizations with different durations of the applied bias. If we adjust and vary the duration of the current pulse, we can realize an MLC with four different states. These states of the FL are illustrated with the blue arrows. The sequential switching procedure of the composite FL is as follows: The torque acting from FL2 on FL1 is opposite to the one acting from the RL, so FL2 switches first, since only the torque of FL1 acts on FL2. After FL2 switches, the torque contributions from FL2 and the RL act on FL1 in the same direction, completing the switching. Since the voltage is maintained, and due to the higher polarization in the middle TB, the magnetization of FL2 is reversed, and the back-hopping effect is realized. The torques from the RL favor the anti-parallel state, while those from FL2 favor the parallel state. However, due to the higher polarization in the middle TB, the torques from FL2 are stronger than those from the RL, so FL1 returns to the initial configuration. This process is repeated for as long as the bias is maintained. If the duration of the pulse is modulated, all four states in Fig. 3 can be realized. A distinction between the two intermediate states can be recognized by the fact that the state with the first FL1 parallel to the RL and anti-parallel to FL2 has a lower resistance than the state with FL1 anti-parallel to the RL and FL2.
The complete analysis of the back-hopping effect in ultra-scaled STT-MRAM cells demonstrated the potential of employing these devices as MLCs with four different states.
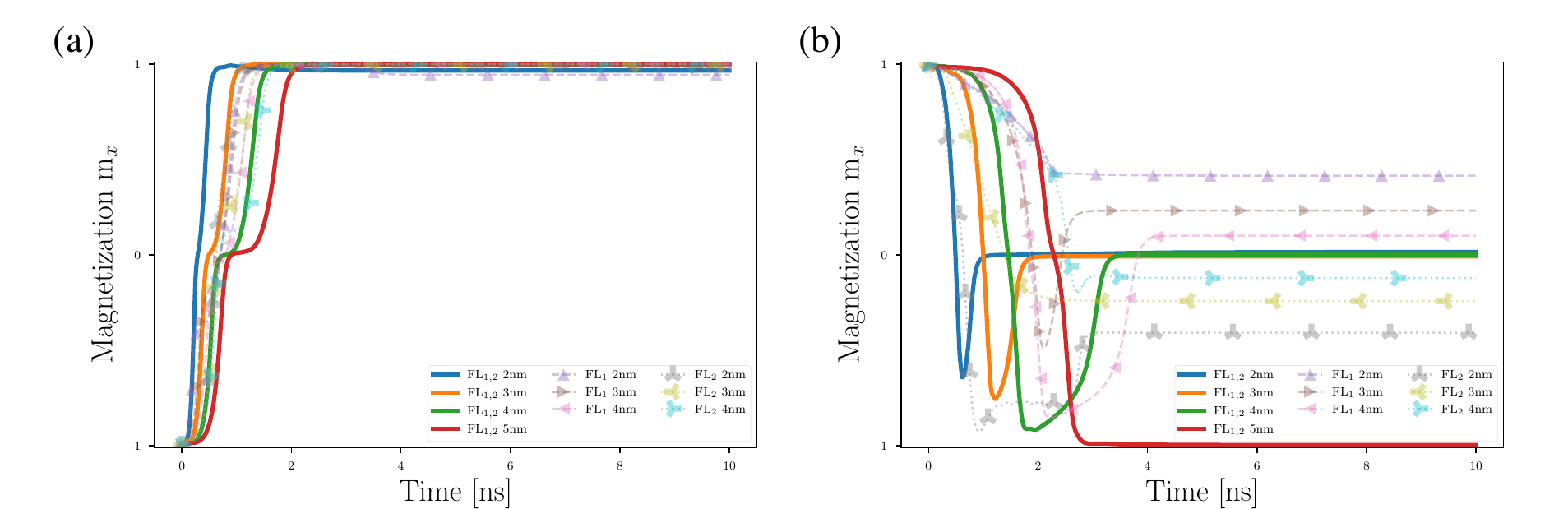
Fig. 1: Magnetization trajectories for the switching from (a) AP to P and (b) P to AP for different combinations of FL lengths. The solid line represents a symmetric configuration, where both FLs have the same length. The dotted line and the dashed line correspond to an asymmetric structure. In the former, the first FL layer length is fixed while the second is varied; in the latter, the second FL layer length is fixed while the first one is varied.
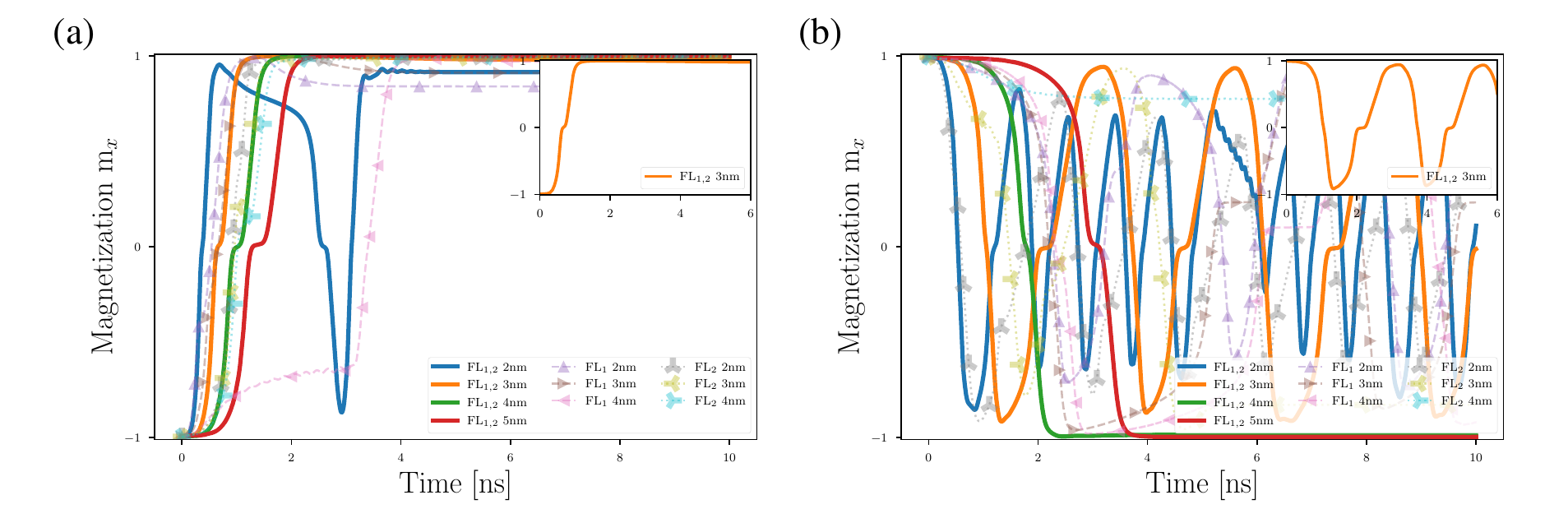
Fig. 2: Magnetization trajectories, when switching from (a) AP to P and (b) P to AP for different combinations of FL lengths, were we applied the same methodology as in Fig. 1, but with increasing polarization of TB in the middle of the FL. In addition, the symmetrical structure with 3 nm thick FLs is highlighted in the inset and has the best prerequisites for an MLC.
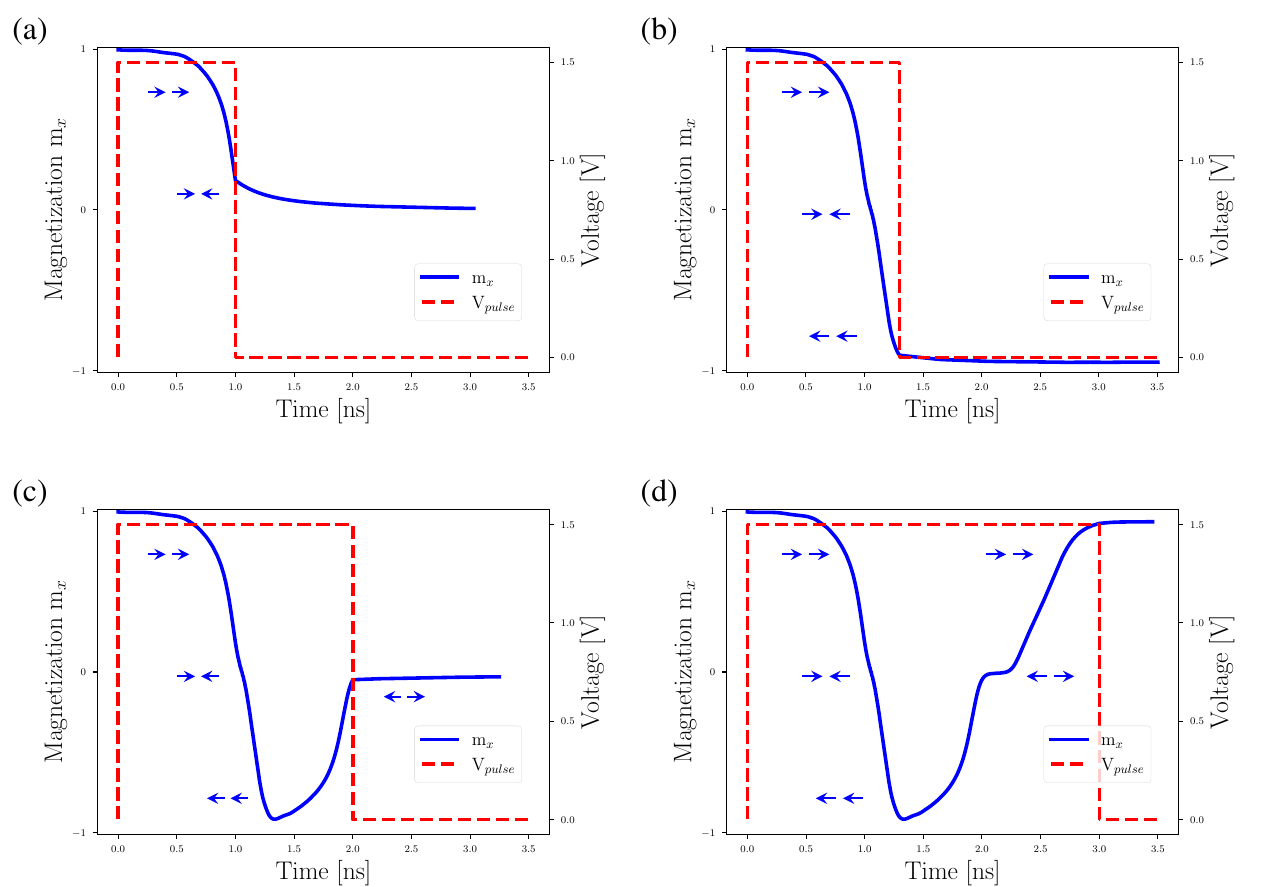
Fig. 3: Magnetization trajectories for a symmetric structure with 3 nm thick FLs, switching from P to AP for different duration of the applied bias. The solid blue line represents the x-component of the magnetization and the red dashed line the duration and amplitude of the bias pulse. The arrows along the magnetization represent the state of FL1 and FL2.